This essay was submitted to Open Philanthropy's Cause Exploration Prizes contest. Edited 01/31/23 to correct a miscalculation in the executive summary.
If you're seeing this in summer 2022, we'll be posting many submissions in a short period. If you want to stop seeing them so often, apply a filter for the appropriate tag!
Executive Summary
Why is Indoor Air Contamination a Problem?
Contaminants in indoor air can damage human health through infectious disease and pollution. Here we focus primarily on harms caused by both endemic and pandemic respiratory pathogens with a brief, incomplete discussion of particulate matter pollution and gaseous pollutants.
Cause | Rough Estimate of Annual U.S. DALY Burden |
Endemic Respiratory Disease | 1.4M[1] |
Pandemic Respiratory Disease | 400K[2] |
Indoor PM2.5 Pollution | 750K[3]-3M[4] |
By our back-of-the envelope estimate of impact, a 25-year campaign that achieved a 90% reduction in the burden of infectious respiratory disease would yield roughly 44 million 20 million DALYs over a 35-year window. (Edit 01/31/23: The initial estimate was incorrectly calculated and caught much later.)
How to Fix Indoor Air Contamination
There are three main ways to reduce indoor air contamination: ventilation, filtration, and exposure to ultraviolet C light (UVC). Ultraviolet interventions can be divided into upper-room UVC (which directs UVC into areas unoccupied by humans) or far-UVC, which does not penetrate human skin and is therefore believed not to be dangerous. Whereas filtration and (to some extent) ventilation can reduce both PM2.5 pollutants and respiratory infection, UVC only targets pathogens. Far-UVC is a new method and while evidence for its safety in humans is promising, it is not yet conclusive.
The effectiveness of air cleaning methods is traditionally measured in air changes per hour or equivalents to air changes per hour (ACH or eACH). For American buildings, 1-2 ACH is currently fairly typical. As explained below, with close-to-presently available technology in congregate settings like schools or offices, ventilation rate increases could reasonably achieve about up to 6 ACH, filtration between 5-10 eACH , upper-room UVC above 20, and far-UVC above 100 eACH. In a room with well-mixed air, each equivalent air change represents a reduction of about 63% in the aerosolized pathogen load, but the relationship of this reduction to disease transmission is not straightforward, and true eACH is heavily influenced by contextual details.
These approaches are complementary, so (if far-UVC proves to be safe) we think it is realistic to achieve a 90-99% reduction in respiratory pathogens present in the air if these methods are cost-effective for government actors.
How Can Funders Improve Indoor Air Quality?
We argue below that directly installing air cleaning equipment is very likely cost-justified for government actors but not for “effective altruist” (EA) philanthropic funders paying for impact competitive with the GiveWell waterline (~$80/DALY). We see an EA cause area to promote indoor air quality (“IAQ”) as primarily an advocacy play that also includes scientific research and market-shaping components.
The size of an IAQ movement contemplated in this document anticipates EA funding of U.S. approaches of roughly $5M-$15M/year over 25 years. We envision IAQ improvements as occurring on an adoption curve affording a range of opportunities for advocacy. These include experimental pilots among exemplary businesses (like FTX or Google), voluntary certifications (like LEED) likely via the industry group ASHRAE , adoption in government buildings (like schools), tax subsidies for implementation in congregate settings, changing building codes for new construction, and building code requirements necessitating installation in existing buildings. Attractive advocacy opportunities likely exist at the local, state, corporate, and federal levels.
Major Sources of Uncertainty in Our Analysis
While clearly aerosol transmission plays a major role in the spread of respiratory disease, other vectors may be significant and therefore reduce the impact of IAQ interventions. Additionally, reducing the amount of pathogen in the air likely does not not correspond to a roughly linear reduction in infection (for example if logarithmically different levels of inhaled pathogen produce similar likelihood of infection). The impact of various interventions is also highly susceptible to implementation details.
Background
Context on This Report: COVID-19 has created a sea change in scientific attitudes towards aerosol transmission of respiratory disease, and the distressing impact of air pollution continues to be documented in greater and greater detail. In this shallow investigation-style report, we explore the case for EA funders to consider efforts to reduce respiratory pathogen burden by improving indoor air quality. We focus on the United States for analytic clarity. (About 1.2 billion people globally live in high income countries, for which deployment should be roughly similar to the U.S.) We do not discuss the benefits to future generations of reducing truly existential biothreats, but those may be substantial.
Existing IAQ Policy and Regulation: The majority of air quality guidance is aimed at chemical/molecular pollutants, with little if any focus on infectious disease.
- World Health Organization: The WHO Guidelines for Indoor Air Quality exclusively references dangerous chemicals and gasses such as benzene, carbon monoxide, and formaldehyde.
- State and Local Government: In the United States, IAQ standards are typically set by individual states. These policies tend to derive from guidelines published by the American Society of Heating, Refrigerating, and Air-Conditioning Engineers (ASHRAE), an influential industry group.
- ASHRAE: The majority of buildings fall under the remit of ASHRAE Standards 62.1 and 62.2, last updated in 2019. They call for varying amounts of ventilation based on occupancy, use, and a constant for area - working out to be approximately 1-2 ACH in residences and offices (though half of studied buildings fall below ASHRAE standards). The standards do not consider airborne pathogens. More stringent requirements can be found for healthcare settings, defined in ASHRAE 170.
- Occupational Safety and Health Administration: OSHA has authority to regulate air in indoor workplace settings. Its regulations tend to be fairly weak, only address particulate and chemical pollutants, and are primarily based upon ASHRAE guidelines. In facilities that are not expected to produce large amounts of pollutants, OSHA demands only a self-certification form, and in environments where pollutants might be more common, only some chemicals are regulated, with a generic requirement for employers to protect against known harms.
- Environmental Protection Agency: EPA is responsible for outdoor air quality but does not regulate indoor air quality; it primarily focuses on greenhouse gasses, radiation, and common hazardous gaseous and particulate pollutants. However, it does provide resources for people seeking to independently improve their indoor air quality.
Federal efforts have been proposed to increase US air quality: the American Pandemic Preparedness Plan (AP3) proposed allocating $3.1B for “next-gen PPE and built environment improvements” (with no indication of the split between the two), but AP3 did not pass, and the funding was not included in the proposed PREVENT bill. Organizations that want to upgrade their ventilation and air purifying systems are encouraged to use funds from the American Rescue Plan and Bipartisan Infrastructure Law to do so, but there has been no specific funding or plan for improving indoor air quality.
What Is the Problem?
Pandemic respiratory disease
Clean air can prevent pandemics, which can have significant benefits. Within the U.S. alone:
- SARS-CoV-2 caused over a million deaths as of this writing;
- An estimated $2 trillion GDP loss occurred just in the first two months of the pandemic.
- Reif et. al. estimate that the COVID-19 pandemic cost 6.62 million QALYs over the course of one year, not including QALYs lost due to restrictions.
The majority of aerosolized respiratory pathogen transmission occurs indoors; in the COVID-19 pandemic it is estimated that likely more than 90% of transmission has occurred indoors and that the odds of transmission are at least 20 times higher indoors than outdoors.
Endemic respiratory disease
The burden of flu varies based on the year and the specifics of the seasonal variants, but it is estimated that seasonal flu causes 34,000 American deaths and represents $11 billion in lost productivity. The WHO estimates that the burden of infectious respiratory diseases in the US was 1.42 million DALYs for the year 2019, providing a useful estimate of the burden of endemic infectious respiratory diseases. Worldwide, the burden of infectious respiratory disease was nearly 114.5 million DALYs for the year 2019.
What Mechanical Interventions Improve Air Quality?
Known effective interventions to improve indoor air quality include HEPA filtering, upper room UVC, and increased outdoor air ventilation. Air quality standards are typically set in terms of air changes per hour (ACH) or clean air delivery rate (CDAR), measured as units of clean air produced per unit time.
Ventilation exchanging outdoor air with indoor directly achieves true air turnover, whereas filtering and UVC impact is measured in equivalent air changes per hour (eACH). The resultant eACH in a room outfitted with filters or UVC lights depends on several different factors, including the quality of the filter or power of the lights, the number and placement of lights or filters, and air mixing. In addition, for UVC application, the pathogen in question is relevant, since the eACH calculation depends on the percentage of pathogens deactivated over the course of the time frame; tuberculosis and COVID-19 are the primary pathogens traditionally used to calculate eACH.
Ventilation: In order for ventilation to be effective, the air entering a room must be of higher quality than the air leaving. Outdoor air is an easy source, provided it is of reasonable quality, meaning this strategy can be less effective in highly polluted areas. In addition, outdoor air generally requires temperature changes to be acceptable indoors, which means expending significant amounts of energy on climate control.
Changing Airflow: In general, ventilation can follow one of three overall strategies - mixing, displacement, and personalized. Mixing ventilation simply adds clean air to dirty air, where displacement ventilation aims to take advantage of the effect caused by pathogens being emitted in a warm plume, combined with the natural heating effect of humans, which likely creates a thermally stratified layer above head height with a higher concentration of pathogens. This process theoretically results in a risk reduction factor of 1.2-2 for influenza. Personalized ventilation gives each occupant a designated ventilation flow, creating a similar risk reduction factor. These strategies, or similar variants, may be effective at reducing pathogen transmission, but data is limited.
Filtration: Filtration involves passing air through a filter, designed to remove some proportion of particles from the air. This method is effective in reducing both pathogen transmission and some indoor air pollution, including PM2.5. In addition, outdoor air can be filtered before being introduced indoors to improve quality. Filters cause a pressure drop - the higher the filtration rate, the higher the pressure drop, meaning more energy is required to move the same amount of air through a building.
Standalone filtration units (graded using the MERV scale, with HEPA filters being the most efficient, removing >99.9% of small particles) have been shown to reduce the exposure to pathogenic aerosols under controlled conditions, with 5 eACH HEPA filtration in classrooms enough to cause a 4-5 fold drop in pathogen dose. Strong evidence exists that increased ventilation has a marked effect on infection rates (and health more generally), indicating a high likelihood of aerosol transmission and supporting the efficacy of ventilation as a general method to reduce prevalence of pathogens. In a model of a 30 person restaurant, with baseline US prevalence, increasing ACH of 0.8 to 12 eACH using HEPA filters averted an estimated 54 COVID-19 infections per year, with a gain of 1.35 QALYs.
Addition of filters to existing ventilation systems in a typical model scenario has been shown to reduce relative risk of infection of influenza by up to 47%, at a total annual cost of $352 for HEPA filters, with MERV 13/14 filters (removing a lower fraction of particles), shown to be nearly as effective at considerably lower costs (total annual cost $156, $119 per unit risk reduction compared with $232 for HEPA).
Ultraviolet Germicidal Irradiation (UVGI)
UVC light can be used to inactivate pathogens via protein/DNA damage, but does not reduce particulate pollution; this is known as Ultra-Violet Germicidal Irradiation, or UVGI. Most spectrums of UVC light are harmful to humans, causing corneal damage within hours, as well as significant skin damage.
Upper-Room UVC: This strategy directs UVC light to the top of the room, so harmful UVC is not passed to humans below. Studies have shown 80% efficacy in TB transmission reduction with guinea pigs exposed to hospital air, with strong evidence demonstrating reduction of various pathogens concentration under laboratory conditions. Models predict between a 1.6 and 3.4-fold decrease of TB infection in a hospital waiting room using lighting with eACH 7.5 and 31.7, respectively. Strong knowledge of the mechanisms of UVC allow creation of predictive models for inactivation ability by pathogen.
Far UVC: Recently, significant interest has grown in far UVC - a narrow band of UVC light which is ionizing enough to inactivate pathogens, but not to penetrate the outer layers of human skin or the corneal layer. In theory, far UVC can be used much more easily in many environments to inactivate pathogens, without harming humans. Far UVC is so recent that it is generally not included in analysis of current interventions, and lacks long-term human safety data.
Far UVC has broad germicidal activity, withlow doses (permitted under current regulations) sufficient to inactivate 90% aerosolized coronaviruses in 8 mins, and 99.9% in 25 mins. Efficacy can vary from pathogen to pathogen, but so far UVC causes no known significant damage to human skin and cell models even at doses significantly higher than required germicidal doses.
Long-term exposure studies in humans and adjustment of regulations could be required for widespread acceptance, and further studies are likely warranted. Given far-UVC (under test conditions) provides 184 eACH at an irradiation level already permitted in the US for 8 continuous hours, no evidence has yet raised concrete safety concerns. Far UVC lamps also generate some ozone, but safe levels/limits of ozone exposure are already regulated by a number of bodies. No standard procedure exists for testing purposes and estimates for production vary widely, so quantification is difficult.
Combining Interventions
A model of American schools suggested that installation of MERV13 filters was more effective than doubling outdoor ventilation from 2 ACH to 4 ACH (but with diminishing returns after 4 ACH), and combining the two was most efficacious. However, in the case of COVID-19, 4 ACH, plus MERV13 filters, plus a 50% reduction in student occupancy was still not enough to bring infection risk below 1% in the US.
Models of varying interventions show that in many spaces, the risk of SARS-CoV-2 transmission can be brought down to 0.1% or lower, and R0 can be brought well below 1. In very general terms, using either 100% outdoor ventilation or HEPA filters can provide an average 27% infection risk reduction, using a standalone HEPA filter can reduce risk by an average of 31%, and UVGI a reduction of 59%. Doubling ventilation rate can reduce infection rate by 37%, but use of displacement ventilation and partitions can reduce risk by 96%.
There are numerous, varied methods to implement these systems. Filtration and ventilation are some of the least expensive interventions, and require no specialist knowledge to install and set up. However, ventilation is likely to be less effective at shorter distances.
Upper-room UVC provides far more eACH per dollar. Ventilation systems draw in less air than can be exposed to an upper-room UVGI system, (in a 9ft tall room, approximately 22% of the air volume can be exposed to UVGI). Natural convection currents increase the fraction of contaminated air drawn up even more, as do simple-to-install low-velocity ceiling fans, but when combined with displacement ventilation, efficacy may drop, as pathogens spend less time being irradiated.
Each air change removes 63% of remaining pathogens, so current pathogens generally do not require eACH this high before diminishing returns reduce cost-effectiveness. As such, when combined with the risks to humans, expertise required to install, and setup costs, UVGI tends to be viewed as an adjuvant to other strategies. However, future pathogens with higher emission rates (ie, highly-infectious pathogens) may benefit from such high turnover, meaning this perceived overkill may be useful when considering the risk of catastrophic future pandemics.
Cost-effectiveness of different mechanical interventions
Nardell (2021) compares the cost-effectiveness of different mechanical interventions, determining that upper-room UV is the best option when comparing it against mechanical ventilation and filtration. Upper-room UV was calculated to produce up to 24 eACH under standard air mixing conditions (ie, air mixing resulting from convection currents and people moving through the room), and was estimated to cost roughly $14 per eACH, making it over nine times more cost-effective than mechanical ventilation. By contrast, three air filters that were compared against mechanical ventilation and upper-room UVC were estimated to cost $100-$300 per eACH, ranging from about half as cost-effective as mechanical ventilation to about the same cost-effectiveness. As a baseline, the model estimates that mechanical ventilation alone provides about one air change for about $135.
As a case study in the cost of upgrading ventilation for a large public space, the Center for Health Security report on school ventilation (Appendix F) focuses on a direct comparison between the cost-effectiveness of ventilation versus the early CDC surface cleaning guidelines. A more comprehensive analysis was prevented by the knowledge gaps in aerosol transmission discussed below. Based on expert interviews, the report estimates that a school would need $6,000 per classroom for upgrading HVAC systems to provide air quality equivalent to about 5 to 7 air changes per hour (ACH). At an estimate of 2.5 million public school classrooms nationwide, the cost of upgrading all schools would be $15 billion (although students in each upgraded school would benefit before all schools were upgraded, so the total outlay is not needed for intermediate benefits). Rothamer et. al. show that increasing airflow in a schoolroom from their measured baseline of 1.34 ACH to 5 ACH reduces the probability of infection by about half.
This report only includes the cost for upgrading systems and running new systems at a basic level, but does not include the costs of post-upgrade energy consumption. If upgrades are done primarily through ventilation, there is substantial added energy consumption, estimated by one expert at a 15-20% overall energy cost increase for 10 eACH throughout a school.
How Much Infectious Respiratory Disease Is Transmitted by Aerosols?
Summary: Relative importance of modes of transmission between pathogens are very poorly quantified – for most common respiratory pathogens (aside from COVID-19 and to an extent, influenza) the data required to make meaningful quantitative predictions does not currently exist. However, at a very rough estimate, we would posit that 90-99% of COVID-19 infections come from aerosol sources (high confidence), between 40-80% of influenza transmission (medium confidence), and 20-90% of the overall disease burden of other common cold viruses (low confidence).
IAQ interventions to prevent disease only act on aerosolized particles. The impact of IAQ on disease transmission is dependent on the fraction of pathogen transmission attributable to airborne transmission. While some diseases, most notably COVID-19, TB, and chickenpox, are widely accepted to be dominantly airborne, most respiratory pathogens have historically been assumed to be primarily driven by large droplet/fomite transmission.
Respiratory pathogens
Covid-19: The vast majority of COVID-19 infection is due to the aerosol route, mostly indoors. Early studies were mostly observational, with a notable early study showing a clear case of aerosol transmission in a restaurant, which, along with increasing evidence of biological plausibility, and evidence that SARS-CoV-1 spread via aerosols, generated calls for the general acceptance of aerosol transmission a few months into 2020.
Influenza: Over the last decades, large amounts of research have been conducted on influenza transmission - but consensus is far from clear. Literature reviews provide convincing evidence of both closer-range/fomite transmission, and transmission via aerosols (though not without divergent opinions). Computational models can also predict dominant aerosol transmission of influenza.
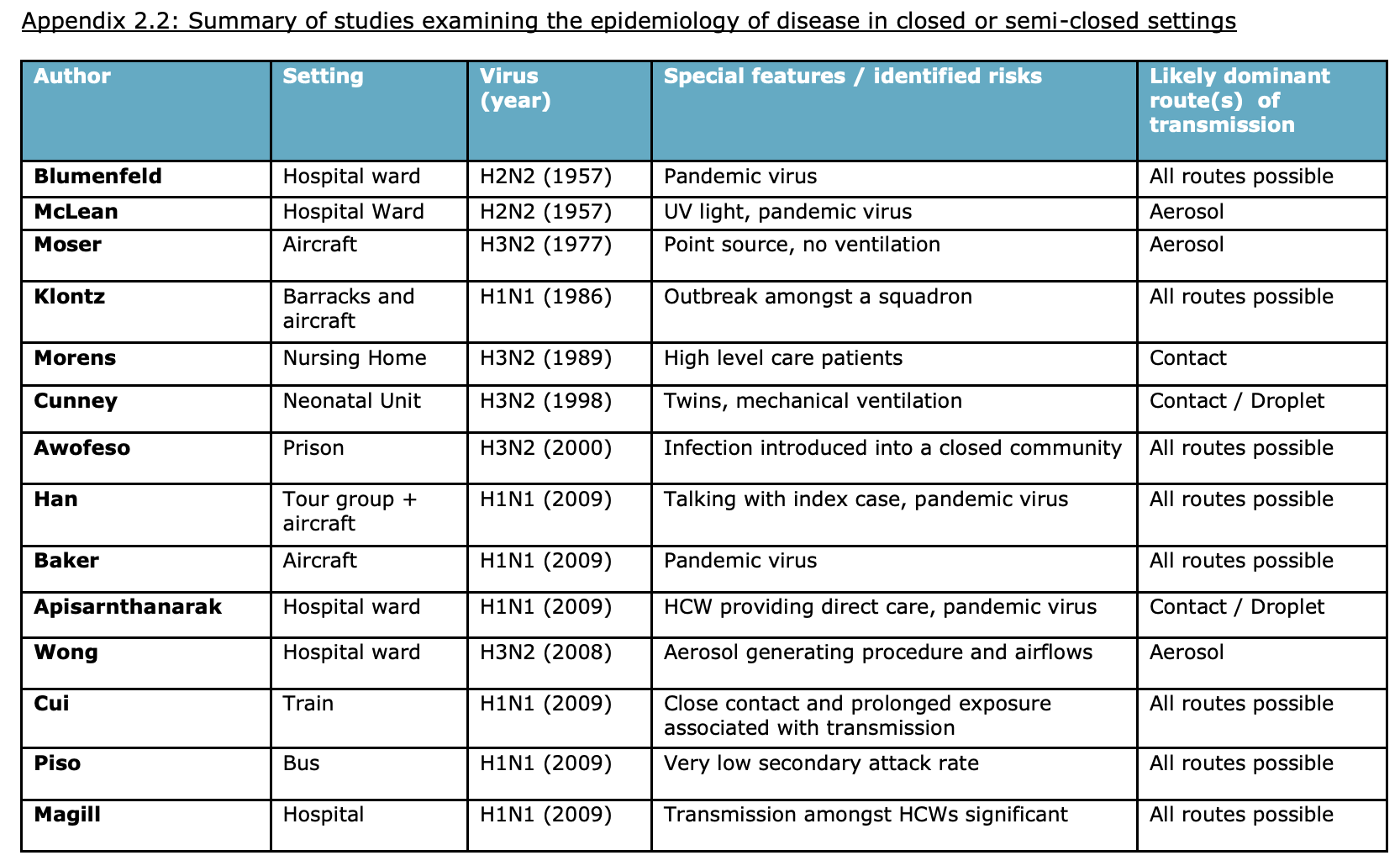
Various real-world intervention and controlled studies have been completed:
- Deployment of upper-room UVGI in a hospital in a 1957 influenza outbreak almost completely prevented transmission, suggesting the vast majority of transmission is airborne.
- Studies in Bangkok and Hong Kong estimated (albeit speculatively) 41-52% of transmission in control arms was via the aerosol route, due to increased symptoms potentially consistent with airborne infection in the intervention households.
- A 2010 study, attempted to cause flu transmission from challenged donors, but (in contrast to an earlier pilot study), found a totally inadequate attack rate, meaning no firm conclusions were drawn. A US-based followup, launching later this year, hopes to improve on this design by using donors with community-acquired infections (removing the possibility of an experimental infection affecting shedding characteristics).
There exists highly convincing evidence of all major transmission routes for influenza. However, it seems reasonable to take as a lower bound 40% airborne transmission (the lowest value in the Bangkok/Hong Kong intervention study), and an upper bound of 80% (based on the success of the Livermore hospital study). Given the high burden of disease, economic costs, and pandemic potential, more research into influenza transmission seems highly warranted.
Common Cold Viruses: From the 1970s to the 1980s, two teams carried out HCTs on rhinovirus, where volunteers challenged with rhinovirus interacted with healthy volunteers. The first, in 1978 in Virginia, found that hand-to-hand transmission is an efficient way to transfer rhinovirus infection, while attempts to cause large droplet and aerosol spread mostly failed. Then in a three-study series running through 1987, a Wisconsin team built a challenge model, found virucidal treated tissues were effective in preventing transmission of rhinovirus, and found that inducing infection via a fomite and large droplet route was ineffective, while measures designed to specifically induce aerosol routes of transmission maintained high attack rates.
The two teams came to two separate determinations of the importance of aerosol transmission. However, according to an expert with inside knowledge of the studies, the Wisconsin studies were more likely to generate accurate results - for example, efforts (not described in the paper) were made to reduce air leakage in the Wisconsin study. Despite these CNE studies being some of the highest-quality research ever performed on pathogen transmission, the results have not caused significant change.
Another study in an Army barracks demonstrated a newer building with a lower ventilation rate was associated with an average of 45% higher risk of common cold infection (typically adenovirus), providing strong evidence that aerosol transmission is important for other common cold pathogens.
In general, despite the more limited range of studies, convincing evidence shows a significantly higher fraction of transmission might be via the aerosol route than historically acknowledged. A reasonable upper bound, therefore, could be high, over 90%.
Limitations
The body of work on this topic is of limited size and quality. Simply isolating a mode of transmission (even before attempting to quantify importance) outside of a highly controlled environment is difficult, and many observational studies suffer from confounding variables. Methods exist to retrospectively model the importance of various transmission routes based on previous data, but suffer from significant gaps limiting use.
Another challenge for both interpretation and usability of data are the development of pervasive errors in the medical literature, significant enough to obviate the results of some studies. Some of the most common are:
- Aerosol size - Many studies assume a binary cutoff of 5um for aerosolized pathogens, and assume that every other pathogen acts ballistically (following a trajectory towards the ground). In reality, particles up to 100um can act as inhalable aerosols, settling towards the top of the respiratory tract. Speaking, singing, or shouting can produce more of these larger particles.
- Close-range aerosol contact - Many studies assume that all transmission that happens at short range (shorter than 1-2m) is due to large droplet transmission, when in fact aerosol transmission can account for up to 90% of exposure at 0.3m, and close proximity can cause more efficient aerosol transmission due to currents generated by body heat.
What Can Funding Accomplish?
Funding opportunities exist in research, market shaping, and advocacy.
- Advocacy: Some presently attractive advocacy projects include: development of an ASHRAE anti-infection standard; recruiting high-status businesses to conduct pilots; improving air quality in schools; and creation of an umbrella advocacy group.
- Research: Attractive research opportunities include: (a) establishing the safety of far-UVC and (b) creating reliable ways to test intervention efficacy, which could include experimental pilot programs or controlled natural exposure challenge studies.
- Market Shaping: Advanced market commitments could drive down the cost of far-UVC and other interventions.
Advocacy
IAQ policymaking occurs along an adoption curve that includes implementation by businesses, voluntary certification codes, retrofitting government buildings (like schools), subsidies for private renovations, building code requirements for new construction, and codes requiring implementation in existing buildings. We see three advocacy opportunities as immediately attractive:
- Corporate Early Adopters: Promoting the piloting of air quality measures by pro-science corporate and educational institutions (like FTX, Google, or Stanford) can generate experimental evidence, define and create precedents for optimal interventions, establish interventions as desirable, and build a constituency for further advocacy. To give an example, a rough highest cost estimate for fitting out Google’s primary campus with upper-room UVGI (a less-studied but high eACH intervention) comes to (based on $2500 per 500ft over 1.1m ft2) $5.5m. While a large sum, this represents less than 0.06% of Google’s annual budget for offices and data centers for 2022 alone.
- ASHRAE Standard: ASHRAE standards for indoor air quality are widely adopted by state and local governments, and ASHRAE has an incentive to promote business for its members. Generating a voluntary ASHRAE standard on clean air quality will de-risk adoption for government and corporate decision-makers.
- School Deployment in Pilot Jurisdictions: Pandemic-proofing schools to prevent learning loss is a timely political goal, and some state and local governments may have COVID relief funds they will need to return if unspent.
Other potentially attractive opportunities are:
- Attaching IAQ Projects to Anti-Recessionary Policies: A perennial problem for anti-recessionary fiscal policy is a lack of “shovel-ready” projects to fund during an economic downturn. Maintaining a warm base of renovation and construction capacity for IAQ retrofits could then be tied into stabilizer legislation that would automatically purchase tens of billions of dollars worth of IAQ installation in the event of a downturn.
- Tax Subsidies: Governments could subsidize installation of basic, known effective interventions in schools, offices, restaurants, and other congregate settings.
- Establishing an Umbrella Organization for IAQ Advocacy: A central organization to manage the IAQ projects above and oversee research and market shaping activities could be attractive to fund if effective leadership can be found.
Market Shaping
An advanced market commitment (AMC) , funded by government, philanthropy, or business, could spur development of a product by committing to a purchase once technology meets certain specifications. One likely use-case could be development of LED-style far-UVC lamps to replace KrCl lamps (currently expensive and produced by only one manufacturer). There may also be relatively expensive products in the upper-room UV and filtration arenae where cost could be reduced by guaranteeing purchases at greater scale, possibly as a way of subsidizing early pilot experimentation.
Research
Far-UVC Safety Testing: Far-UVC is a potentially transformative intervention, and studies to develop a safety record sufficient for wide use in humans should be a high priority. Studies have already been successfully conducted on realistic 3D skin models, with intense monitoring for damage, and some longer-term studies on mice made deliberately susceptible to tumors. Interventions of a similar risk have been proposed based on the evidence of models. In-human longer-term studies could be feasible on a dedicated population (possibly an office block), with monitoring for early signs of damage, combined with an early efficacy study.
Valid Efficacy Models: Creating a way to experimentally test the efficacy of various IAQ interventions will be a necessary component of engendering and optimizing implementation over the long-term. One model to do so is the idea described above of randomizing experimental pilots in early adopters.
CNE studies: Another approach is to utilize controlled natural exposure (CNE) studies, which are a version of human challenge studies where uninfected “recipient” volunteers are exposed to infected “donor” volunteers. Despite their ability to provide some of the highest-quality, cleanest quantitative data of aerosols, transmission routes, and interventions, they are uncommon, with only two large-scale studies in the last two decades - one in 2010, finishing with an attack rate too low to be of use and one additional study planned over the next five years.
We think that exploration of CNE studies stands to be a valuable research contribution requiring a high level of cooperation between fields.That said, these studies are still in their infancy; using them to experimentally test intervention efficacy may require significant investment on the order of tens of millions of dollars, which may not be cost-justified from an EA perspective.
To provide a rough estimate of the impact of a widespread air quality campaign in the US, we made some basic assumptions about the timeline and possible impact of a campaign, and compared the result against a counterfactual baseline. You can see our calculation here and input new assumptions to see how they affect a campaign’s impact.
Our process
The drafting of this report was funded by an FTX Foundation grant focused in part on the evaluation of controlled natural exposure challenge studies. We initially became interested in indoor air quality as a result of researching ways to mitigate airborne respiratory virus transmission, since such viruses seem particularly dangerous in terms of causing deadly pandemics. In the course of our research, we found that there were other potential benefits from improving indoor air quality.
We started with a literature review focused on flu transmission and then interviewed several experts in aerosols transport. After these conversations we returned to literature review, guided by the interviewees’ recommendations.
- ^
Per WHO estimate.
- ^
Assumes 10 million DALYs lost to COVID in U.S. and that a COVID-size pandemic happens every 25 years.
- ^
- ^