By Mike Cassidy and Lara Mani
Ord 2020, and others have suggested that the existential risk posed by volcanoes is the largest of the potential ‘natural’ catastrophes - 100 times that of asteroids and comets combined. As volcanologists we wanted to delve a bit deeper into the x - risks and also global catastrophic risks from large explosive eruptions, adding insight from the latest volcano and climate science, including challenging some of the assumptions that have been put forward so far. Significant global impacts from large explosive eruptions will be described, which have a ~1 in 6 probability of occurring this century.
Introduction
I’m Mike and new to EA world, having just been introduced to it early this year from a friend in Oxford and having thoroughly enjoyed reading The Precipice, the super-eruption part sparked my interest. I’m a senior research fellow based in the earth science department at the University of Oxford, and specialise in volcano research. I research a range of different aspects in volcanology, but principally I aim to understand what influences the explosivity of eruptions and how best to forecast them. Lara works for the Centre for the Study of Existential Risk (CSER) at the University of Cambridge and has been thinking about extreme volcanic risk scenarios since joining the centre in January 2020. In particular, Lara has been exploring the systemic nature of volcanic risk from the view point of cascading and compound risks.
The idea of this post here is to provide some thoughts that might interest the EA community from a different perspective. I am also slowly catching up with the existential risk literature (this is partly time, but also because I wanted to provide a fresh insight initially without thoughts being diluted by later reading), so if you know literature that may be connected with some of the themes we cover, then please let us know. This really is the start of us thinking about this topic and as you’ll see there’s lots of uncertainty and we’d welcome some thoughts and questions about this generally to assess the gaps going forward.
Challenging assumptions and why we think the current risk may be underappreciated
The focus of impacts from large explosive eruptions has so far focussed on the initial cooling effect (which could be substantial), but the effects are far more varied than this, which we’ll discuss in later in this article and in our modern, connected world, perhaps means that we could more vulnerable to these. In this post we aim to challenge two notions that have been put forward in the existential risk community previously:
1) That the risk from volcanic eruptions is ‘natural’ and hasn’t changed/won’t change going forward
2) That humans have survived 2000 centuries therefore the natural risk must be low
The first assumption is challenged by recent studies which show that anthropogenic climate change increases the climatic cooling effect of large magnitude explosive eruptions (potentially by as much as 60%; Aubry, 2021 & Fasullo et al 2017); climate change itself also may increase the likelihood of triggering eruptions through glacial retreat and sea level change. Furthermore as we explore below, the more extreme climatic effects are not always attributed to the largest magnitude eruptions, in other words, you don’t need a ‘supereruption’ to cause global climatic impacts. All these factors, along with new ice core records showing large explosive eruptions are more common than values Ord 2020 used, mean that our current base rates for global catastrophic risk and existential risks might be higher than initially appreciated.
The 2nd assumption seems to be more based on human extinction, and negates the impact on civilisation collapse/failed potential part of the existential risk definition. Since human civilisation began (~10,000 years ago) a volcanic eruption of magnitude 8 or above has not occurred, and thus this possibility remains untested. (Though smaller magnitude eruptions may have led, directly or indirectly, to the collapse of smaller civilisations (e.g. Minoan, Maya, Romans (Druitt et al., 2019; Nooren et al., 2017; McConnell et al., 2020), as well as the extinction of other hominids, e.g. Homo floresiensis, and potentially Neanderthals; but there is much debate about this (Westaway et al., 2007; Fitzsimmons et al., 2013)). Furthermore, a future volcanic winter may be more extreme than our previous large explosive eruptions due to anthropogenic climate change. The range of different impacts that volcanoes may induce (aside from climatic), i.e. ozone destruction, reduction in rainfall, further biodiversity collapse/environmental damage (already in decline), widespread blockages of maritime trade, destruction of communication, and technology, financial losses, may lead to mass shortages of food, water and energy resources. All of which may have considerable impacts to our interconnected global civilisation, and may lead to collapse, especially if they occur in certain geographic regions or pinch points (Mani et al., 2021).
Putting aside large igneous provinces (massive outpourings of lava that cause various mass extinctions in the geological record), large magnitude explosive eruptions are highly unlikely to trigger human extinction from their impact alone, but below we put forward some ideas about how eruptions may lead to failed potential/civilisation collapse themselves or act as existential risk factors. We won’t provide a new estimate for probability of existential risk from volcanic eruptions from Ord’s 1 in 10,000 chance this century estimate, as we think this requires more work to really understand how the consequences of eruptions could lead to civilisation collapse/failed potential, but that is our eventual aim, and we’d appreciate any thoughts that the community have on this.
Context, definitions and current considered threat from large volcanic eruptions
Ord 2020, as well as the previous scientific community (e.g. Sparks et al., 2005) suggest that the main existential risk posed by large magnitude volcanic eruptions is their ability to perturb our global climate. This can occur in two ways: 1) through explosive eruption of volcanic ash and sulfur into the upper atmosphere, which in doing so reflects solar radiation back into space and results in global cooling (much like nuclear winter, although cooling is instigated by black carbon in the atmosphere, whereas sulfur aerosols is responsible for volcanic-derived cooling), 2) or through voluminous eruptions of lava flows (known as Large Igneous provinces), which release massive amounts of CO2 (and sometimes other greenhouse gases) driving global temperatures much hotter (akin to anthropogenic climate change).
Generally we’ll try to avoid using the terms ‘supervolcanoes’ (as this is a bit of a misnomer as even large volcanoes like Yellowstone may often produce small eruptions), or Supereruptions (eruptions of over 1000 km3 of volcanic material), and instead will use ‘large magnitude eruptions’. This is because the extent of a volcanic eruption’s climatic effect is not only determined by the volume of material that erupted, but various factors which I’ll talk about below (some of which we’re still finding out).
We’ll refer to large magnitude eruptions as anything from magnitude 7 and above, using the volcano magnitude scale, as such large magnitude eruptions have been shown to have global effects (Newhall et al., 2018). The volcano magnitude scale is logarithmic and refers to the mass of material erupted ranges from 1-9, with each magnitude erupting 10 times the mass. The Icelandic volcano that grounded European flights in 2010 was a 4, Krakatau 1883 eruption (the explosion of which was heard 3000 miles away) was a 6, and generally ‘Supereruptions’ are magnitude 8+.
Magnitude | Last eruption & date | Recurrence rates rock record (years) | Recurrence rates ice core record (years) |
7 | Tambora 1815 (Indonesia) | 1,200 (95% CI: 680 - 2100) | 625 |
8 | Taupo, New Zealand (26,000 years ago) | 17,000 (95% CI: 5,200, 48,000) | 15,000 |
9 | Toba, Indonesia (74,000 years ago) | (95% CI: 60,000 to 6,000,000) | N/A |
9+ (Large igneous provinces) | Columbia river basalt (Northwestern US) 16-6 million years | 20-30 million | N/A |
The table above provides information of the last large magnitude eruptions from the rock and ice record, though eruption deposits can be missing from the rock record due to erosion. Some recurrence rates are given from statistical models Rougier et al., 2018 (for Mag 7, 8 & 9). The Ice core record is not able to measure the magnitude of the eruption directly, but can ascertain the recurrence rate of eruptions with a similar sulfur content (i.e. climatic effect) to a magnitude 7 (Tambora) and magnitude 8 (Taupo) (Lin et al., 2021). Note the magnitude 8 eruption recurrence rate for ice cores is only based on 3 eruptions. The recurrence rates from magnitude 9 (i.e. Toba) are especially poorly constrained, but values of 70-80,000 years are given in Papale, 2018. And the recurrence rate from large igneous provinces are from Ernst, 2021.
In this article we won’t delve into large igneous provinces which are responsible for several of the largest extinctions in geological time, these are very rare and the mechanisms that lead to existential risk are considerably different (See EA post: Pangea: The worst of times). Though if a large igneous province eruption were to occur (chance of ~1 in 300,000 this century), the extreme temperatures that result from massive CO2 emissions (exacerbated by anthropogenic emissions) may well lead to human extinction. Instead, in this article we’ll mostly cover the effects from large magnitude explosive eruptions, which occur more frequently than large igneous provinces. If we use the recent ice age estimates this suggests that the combined probability of an eruption of magnitude 7 and above occurring is ~1 in 6 this century.
Climate effects not always proportionate to size of eruption and other uncertainties
As alluded to above, the magnitude of the eruption (more powerful/more erupted volume), does not always scale linearly with its climate effect. Instead the climatic effects from explosive eruptions are controlled by the sulfur content of the magma, geographic location, time of year, eruption plume height, halogen contents, height of the tropopause and other ocean-ice-atmospheric feedback cycles (lesser understood). For example, despite the more than 100 times more material erupted at Toba (mag 9) than Rinjani (mag 7), their total sulfur contents (one of the main controls on volcanic cooling) are thought to be of similar order of magnitude (100’s Tg) (though our ability to estimate the sulfur content from previous eruptions has considerable uncertainties currently). This means that lower magnitude eruptions (7-8) (which occur more frequently), can lead to a similar degree of climatic forcing as magnitude 9 eruptions. This is why we consider the record from ice cores (Table 1), which record approximate sulfur contents, to be a more reliable estimate of the recurrence rate of the largest climate-altering eruptions.
The big uncertainty in the climatic effect of large explosive eruptions is feedbacks that sometimes (but not always) occur with the climate system, which can lead to decadal and even millennial scale climate cooling, these are especially hard to replicate with our climate models currently, as they involve modelling ice sheets, ocean circulation and atmospheric changes (Baldini et al., 2015; 2018). One example is the mini ice age (from 1300 to 1850, where temperatures dropped enough for the Thames to freeze over) which was thought to be triggered by the magnitude 7 eruption Mt Rinjani (aka Samalas) in 1257 and sustained by smaller eruptions (Miller et al., 2012). The Samalas eruption itself is thought to have led to more than 10,000 deaths in London alone due to famine the year following, as well as more widespread, but poorly understood effects in the northern hemisphere (Newall et al., 2018).
Currently it’s unclear why some eruptions trigger longer timescale (>10 years) cooling effects, while some do not. This may be in part controlled by the state of the climate at the time of the eruption. Eruptions that occur when the climate is in a delicate state (i.e. tipping towards an ice age) a large eruption may push it over the edge. Note that this is relevant for those studying nuclear winters too and requires more investigation. In an ‘anthropogenic climate change world’ (aka Anthropocene), it turns out that the climatic cooling effects from large explosive eruptions may be more pronounced.
Impact of climate change
Two recent papers have suggested that the current state of the climate may increase the cooling potential of large explosive eruptions in the tropics, as a result of changes to atmospheric and oceanic circulation (Aubry et al., 2021; and Fasullo et al., 2017). Brewer-dobson circulation (a process where warm tropospheric air rises into the stratosphere, moves towards high latitudes and sinks) is now much faster due to climate change, which means it can transport volcanic ash and sulfate aerosols to higher latitudes faster leading to 30% more surface cooling (Aubry et al., 2021). Along with modifications to ocean stratification and near surface winds, this effect could lead to as much as 60% more cooling in the future (Fasullo et al., 2017). This means that lower magnitude eruptions (e.g. mag 7), which are far more common than magnitude 8 or 9, may lead to more significant and abrupt climate disturbances than have been seen in the past. For instance the 1257 eruption of Mt Rinjani led to a 2.5° C global drop in global temperatures (Liu et al., 2020), in the current climate may lead to a ~4° C global cooling. This means that the base rates in the past for climate changing eruptions (and assumed survival, based on past human existence), might not be the same, and may greatly increase this probability of climate changing eruptions.
Another effect is climate change’s effect on the eruptibility of volcanoes. Climate change melts glaciers, ice sheets, increases rainfall, and modifies the sea level, all factors that may alter the stress fields around volcanoes, removing overlying weight on a volcano may promote magma to ascend to the surface. Places like the Andes, Alaska, Kamchatka and Iceland may become more volcanically active, and may increase the potential for large explosive eruptions. This is something we have seen in the geological record (Swindles et al., 2018) and also in recent ice core records, which show an increase of large eruptions after the deglaciation 16-9 ka (Lin et al., 2021). However the biggest uncertainty is currently based around the timescales of the volcanic response following a warming period (estimates range from years or centuries). So it is currently difficult to assign a different eruption frequency based on current climate change.
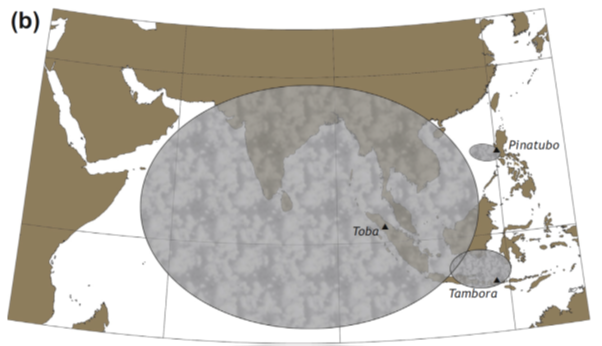
Map of Asia showing areas that were buried in 5 cm of ash, from Pinatubo (mag 6), Tambora (mag 7) and Toba (Mag 9) (from Self et al 2015). Note: new estimates of the ash deposition from Toba moves the ash cloud more towards the west, across the Indian ocean.
What other effects (as well as cooling) can large explosive eruptions inflict?
In the table below we collate the various effects from eruptions both more proximal to the volcano and globally and over different timescales (collating information from Self, 2015 and Newall et al., 2018). These are ordered from the most proximal effects first with global and longer-lived effects later. Not all of these will necessarily occur with every large explosive eruption (e.g. landslides and Tsunamis) and it is important to note that large explosive eruptions may continue for months perhaps years (with sporadic activity), which may make some of these more persistent hazards. Apologies for brevity of information in this table- (we could write essays in themselves for each of these), this is simply to lay out the range of different effects resulting from large explosive eruptions, which is far beyond just the global cooling often cited, and as this is still a young science, there are most certainly unknown unknowns and we’re still learning about other potential impacts.
Phenomena | Immediate effect | Cascading impacts | Timescale |
Pyroclastic density currents (aka pyroclastic flows) | Area affected: <100 km >500° C hot flows with gas, ash and debris flowing like an avalanche over the land. >90% human & animal mortality rate. Buildings destroyed and land buried in >10s metres of rock/ash. Fires on a local scale Damming of rivers may lead to mass breakout floods. May cause tsunamis if they enter water (see mass landslides below) | May bury and destroy most infrastructure (electricity, gas/oil lines, communication lines, transportations networks, and submarine cable connections.) Drinking water contamination. Destruction of agricultural land for years. Refugees: may lead to a large number of people requiring relocation | Length of the eruption (days to months, some very large eruptions may occur intermittently over several years (v uncertain). |
Mudflows (Lahars) | Area affected: 100-1000s of km When ash & rock mixed with water to cause powerful flows. The effects are very similar to pyroclastic flows | As above, but the effects occur on a larger area, anywhere where ash falls (see figure above) and there is rainfall. | Secondary mudflows- rainfall on ash/rocks - years to decades after an eruption |
Pumice and Ash fallout | Area affected: 1000s of km Widespread environmental damage (especially <100 km). Widespread building roof collapse (especially with rain). (see figure above for extent of ash cover). Aviation-risk to flying aircraft. The impact to air and other transport may hamper relief efforts for weeks and limit evacuation
| Destruction of infrastructure, such as power generation (i.e. hydroelectric and nuclear plants) and power transport (i.e. powerlines susceptible to ash loading and electrostatic effects) blocking of shipping lanes if at sea, effect on marine life. If entered into the upper atmosphere, it may block communications with satellites. Drinking water, sewage blockage (and subsequent epidemics). If ash blanket is widespread over land, may increase albedo & enhance cooling (Jones et al 2005) and (depending on thickness) damage agricultural land for years. Stoppage of aviation for months if not more will impact supply chains and financial losses | Across the length the eruption, but ash may remain for months-years |
Air pollution (ash and gas) | Area affected: 1000s of km Increase in particulate matter (10 and 2.5 microns) as well as harmful gases and acids (HCl, HF, H2SO4) which may form dry fogs both impacting human and animal health in a myriad of ways (especially respiratory-related). Chemical etching effects of aerosol particles on aircraft engines and instrumentation | Areas like India, China with poor air quality currently may be most vulnerable. May impact crop production and environmental damage 1000s km away- via acid rain fallout Fills up hospitals over large distances. | <5 years (worse effects closer to the source of the eruption, but highly dependent on wind patterns) |
Mass landslides & Tsunamis during caldera collapse | Area affected: Widespread coasts across the world Often associated on large ocean island after large explosive eruptions (e.g. Tenerife) (Hunt et al 2018) If on land, burial and destruction of a large area, destruction to infrastructure | Large tsunamis akin to the Boxing day tsunami, affecting coastlines 1000s of km away. Ecosystem damage for years to decades, with resulting impacts of fishing industries.
| Across the length the eruption with destabilising effects potentially lasting for years post-eruption. |
Ozone destruction | Area affected: Global Greater rates of UV radiation, reduction of plant growth (Osipov et al 2021). | Humans may be unable to go outside for long periods of time. Affects food security | <5 years |
Rainfall reduction | Area affected: Global Up to 25% global reduction modelled in the case of Toba eruption, but highly uncertain. | Water shortage in some areas, crop growth and food production. More intense in some places compared to others, reduction especially in tropics, Asian and African monsoon. | <5 years |
Global cooling | Area affected: Global High sulfur eruptions may cause negative radiative forcing causing abrupt 3-5° C cooling (enhanced by current climate change) | Food scarcity Resource shortages Mass migration Global financial markets | <5 years |
Climate feedbacks | Area affected: Global May enhance cooling in some places (warming in other places), via ice sheet growth, disturbance to ocean circulation, ash albedo increase, e.g. reduced heat flow to the Arctic ocean, El Nino etc) | Loss of food, mass migration/refugee crises. Paradoxically, high latitude warming (in the hemisphere opposite the eruption), ice sheet collapse, and catastrophic sea level rise. (Baldini et al 2015) | 10-1000 years (dependent on ‘state of climate’ at the time) |
Eruptions as global catastrophic threats and existential risk factors
As shown in the table above, large explosive eruptions have a range of different effects and impacts which in themselves represent clear global catastrophic risks, leading to extensive loss of life. The extent of fatalities will depend not just on the magnitude of the eruption, but principally the location of the eruption (e.g. whether ash fall occurs over oceans versus land), and proximity to populous and/or vulnerable populations, and infrastructure, with cascading impacts far beyond the direct impacts of ash fall for instance.
In terms of the global impacts from large explosive eruptions, the closest analogy is nuclear war scenarios (though this does not capture all the hazards in table above), one such study modelled the food production resulting from a limited nuclear war between Pakistan and India, leading to a global cooling effect of 1.8° C for 5 years and 8% reduction in precipitation (Jagermeyra, 2020). The impact on crop production in those 5 years was substantial: 20 to 50% losses above 30° N, or 11% globally. These temperature & rainfall reductions are typical of the consequences of a magnitude 7 volcanic eruption like Rinjani (especially due to changes in atmospheric circulation), which have a ~1 in 7 chance of occurring this century. This does not include the effects of stratigraphic ozone loss from eruptions that could incur on crop production. Furthermore, larger magnitude (8-9) or high sulfur emitting eruptions may double the cooling and precipitation reduction in the Jagermeyra (2020) scenario, as well as considerable unpredictability of climate feedbacks, which may sustain cooling for decades or longer. As a result, we suggest that global famine poses a real risk from a large explosive eruption.
In some regions access to drinking water may become scarce if contaminated by ash or acid rain, if water purification systems are damaged, or for populations relying on rainfall for drinking water (these effects are highly unknown). Together with fatal impacts close to source as well as globally scarcity of resources like food and water critical life systems, we believe volcanoes pose undeniable global catastrophic risks.
We also believe that large eruptions may serve as existential risk factors, making the following x-risks in Ord (2020) more probable, such as a) environmental damage (many ecosystems would be disrupted), b) natural pandemics due ecosystem and climate disruption, with large volcanic eruptions associated with historical plagues (see Fell, 2020) c) nuclear war and d) engineered pandemics, due to increased likelihood of social unrest or global power war via mass migration to find habitable ‘warm regions’ (e.g. Xu et al 2019), and global resource limitation (food, water, energy etc).
Societal vulnerability to extreme volcanic risk
In this piece so far, we have explored a hazard-centric approach to consider extreme volcanic risk scenarios but one component of the risk equation that is often neglected in the existential risks literature, is a consideration for the systemic nature of volcanic risk. In a recent publication in the journal Nature Communications, we began to explore some of the scenarios which may present themselves if a volcanic eruption were to occur in proximity to global critical infrastructures networks or systems.
Building on the works of Avin et al., (2018) and Liu et al., (2018), we considered extreme volcanic risks through the lens of ‘existential vulnerabilities’. Increased globalisation and demand for goods and commodities now means our societies are reliant on a myriad of systems and networks supplying to support their continued development. These systems and networks, such as maritime shipping routes, submarine communications cables and transportation networks, often converge in narrow geographical localities, such as the Strait of Malacca or the Strait of Luzon. Often, these regions of systems convergence coincide with regions of active volcanism – ‘pinch points’ (Mani et al., 2021).
The table above demonstrates how certain volcanic hazards can damage or destroy critical infrastructures and systems. Electrical grids, water supply networks, power stations, pipelines, transportation routes, ports and airports are all susceptible to damage from volcanic hazards such as volcanic ash fallout, pyroclastic flows or mudflows. In some areas, the impacts may only be felt locally or regionally, but in areas where the criticality of such infrastructures increases, so too does the possibility of a globally felt repercussion. For example, a volcanic eruption in the Mediterranean from volcanic centres like Mount Vesuvius (famous for the AD79 Pompeii eruption), Santorini (linked with the collapse of the Minoan civilisation) or Campi Flegrei could result in ash plumes closing European airspace (as seen during the 2010 Eyjafjallajökull, Iceland eruption). Additionally, a volcanically induced tsunami (such as happened during the 350 BCE Minoan eruption of Santorini) could rupture maritime shipping routes and damage submarine cables. The knock on implications would be felt globally, with disruptions to just-in-time supply chains and trade resulting in shortages of essential goods and commodities for months (or potentially longer) in the aftermath. The feedback loops from such an event would impact upon the global financial markets, oil and food prices, potentially tilting us towards a global financial crisis.
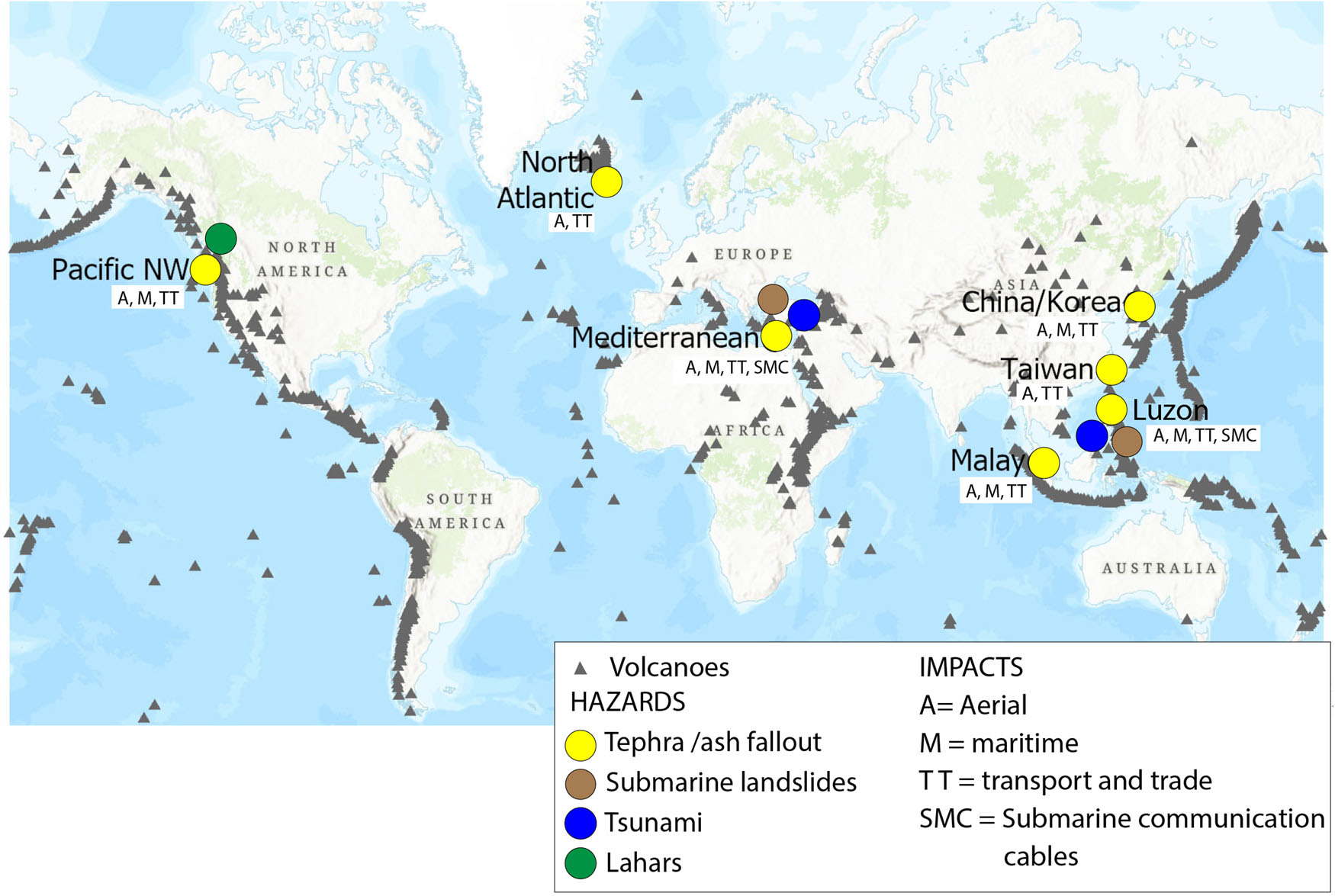
Seven global ‘pinch points’ from Mani et al. (2021), demonstrating areas where a convergence of global critical systems and infrastructures intersect with regions of active volcanism. The seven global pinch points include: the Pacific Northwest, the North Atlantic, the Mediterranean, Strait of Malacca, Strait of Luzon, Taiwan and the South China Sea.
Of significance to these scenarios is that a large magnitude eruption (mag 7-9) is not necessary to instigate the cascading global consequences. Certainly, lower magnitude volcanic eruptions (mag 3-6) are capable of producing the volcanic hazards such as those mentioned in the above table, which can interact with our critical infrastructures and systems. Essentially, by converging some of our societal weaknesses in narrow geographical regions and by prioritising efficiency over resilience in our global critical infrastructures and systems, we have proliferated our own vulnerability to volcanic eruptions and manufactured a new landscape for global catastrophic risks. It may be feasible through these mechanisms above, that large explosive eruptions (and their cascading impacts) pose an existential risk via civilisation collapse, especially if they occur close to global pinch points, but this is an open question that requires further understanding.
Conclusions and the future
We hope this article provides some more context and information regarding the potential ways humans can be affected by large explosive eruptions, which are not restricted to their global cooling impact (though that could be substantial). We know that the effects of these large explosive eruptions may promote more eruption-induced cooling due to climate change and that eruptions could become more frequent, but the latter is more uncertain. It is highly unlikely that a large magnitude explosive eruptions (7+) would directly cause human extinction as seen from our survival from Taupo (mag 8) and Toba (mag 9) eruptions, especially due to our more widespread and adaptable nature. However a human survival rate based on so few large magnitude eruptions is not representative (e.g. the quirks of the Toba eruption; Black et al., 2021) and plays into the ‘near miss’ bias, because another eruption occurring in modern times at a ‘global pinch point’ location may have drastically different impacts. What is clear though is that large magnitude eruptions (mag 7+), with a cumulative probability this century of ~1 in 6, are a demonstrable global catastrophic threat and through food and resource impacts would lead to mass global suffering, as well as acting as a not insignificant existential risk factor to x-risks such as environmental damage, pandemics and nuclear wars. What is unclear at the moment is if large explosive eruptions can lead to existential collapse via civilisation or environmental collapse thus leading to the failed potential of humanity. There are certainly many ways that large eruptions may affect trade, finance, electricity, energy, communications, food and water resources, that could indirectly lead to civilisation collapse (highly uncertain currently- but we welcome your thoughts).
Modern volcanology is a young science (~1960s), with still so many unknown unknowns, as a result we (volcanologists) are largely unprepared for such eruptions (Papale and Marzchochi, 2019). We are still a long way off from coordinating our efforts to understand and mitigate these extreme hazards, especially compared to the planetary defence community (for reasons we can explain in a follow post, if of interest). Some may well ask, apart from preparing for the hazards themselves, is there anything we can do to interfere with them to lessen their impact somehow? It’s topic that that has been received some attention from other disciplines (Dekenberger and Blair, 2018, Wilcox et al., 2015), but it has largely ignored by volcanologists, however we think that this may all change in the next few decades due to recent scientific developments which may drill into volcanoes to tap their fluids rich in ‘critical metals’ (e.g Copper and Lithium) that are needed for green technologies, as well as so called ‘supercritical’ geothermal energy. Some of this is a long way off yet, but there’s lots that may change in the long-term future of volcanoes, so watch this (volcanic) space.
We welcome any feedback or comments, questions you have about this.
References
Aubry, T. J. et al. (2021) ‘Climate change modulates the stratospheric volcanic sulfate aerosol lifecycle and radiative forcing from tropical eruptions’, Nature Communications. Springer US, 12(1). doi: 10.1038/s41467-021-24943-7.
Avin, S., Wintle, B. C., Weitzdörfer, J., Ó hÉigeartaigh, S. S., Sutherland, W. J., & Rees, M. J. (2018). Classifying global catastrophic risks. Futures, 102, 20–26. https://doi.org/10.1016/j.futures.2018.02.001
Baldini, J. U. L., Brown, R. J. and Mawdsley, N. (2018) ‘Evaluating the link between the sulfur-rich Laacher See volcanic eruption and the Younger Dryas climate anomaly’, pp. 969–990.
Baldini, J. U. L., Brown, R. J. and McElwaine, J. N. (2015) ‘Was millennial scale climate change during the Last Glacial triggered by explosive volcanism?’, Scientific Reports. Nature Publishing Group, 5, pp. 1–9. doi: 10.1038/srep17442.
Black, B. A. et al. (2021) ‘Global climate disruption and regional climate shelters after the Toba supereruption’, pp. 1–8. doi: 10.1073/pnas.2013046118.
Denkenberger, D. C. and Blair, R. W. (2018) ‘Interventions that may prevent or mollify supervolcanic eruptions’, 102(January), pp. 51–62.
Ernst, R. E. (2021) ‘Large Igneous Provinces’, Encyclopedia of Geology. Academic Press, pp. 60–68. doi: 10.1016/B978-0-12-409548-9.12528-X.
Fasullo, J. T. et al. (2017) ‘The amplifying influence of increased ocean stratification on a future year without a summer’, Nature Communications, 8(1). doi: 10.1038/s41467-017-01302-z.
Fell, H. G. et al. (2020) ‘Volcanism and global plague pandemics : Towards an interdisciplinary synthesis’, 70, pp. 36–46.
Fitzsimmons, K. E. et al. (2013) ‘The Campanian Ignimbrite Eruption: New Data on Volcanic Ash Dispersal and Its Potential Impact on Human Evolution’, PLoS ONE, 8(6), p. 65839. doi: 10.1371/journal.pone.0065839.
Hunt, J. E., Cassidy, M. and Talling, P. J. (2018) ‘Multi-stage volcanic island flank collapses with coeval explosive caldera-forming eruptions’, Scientific Reports, 8(1). doi: 10.1038/s41598-018-19285-2.
Jagermeyr, J. et al. (2020) ‘A regional nuclear conflict would compromise global food security’, Proceedings of the National Academy of Sciences of the United States of America. National Academy of Sciences, 117(13), pp. 7071–7081. doi: 10.1073/pnas.1919049117.
Jones, M. T., Sparks, R. S. J. and Valdes, P. J. (2007) ‘The climatic impact of supervolcanic ash blankets’, Climate Dynamics, 29(6), pp. 553–564. doi: 10.1007/s00382-007-0248-7.
Lin, J. et al. (2021) ‘Magnitude , frequency and climate forcing of global volcanism during the last glacial period as seen in Greenland and Antarctic ice cores ( 60-9 ka )’, (August), pp. 1–45. (PRE-PRINT)
Liu, B. et al. (2020) ‘Global and Polar Region Temperature Change Induced by Single Mega Volcanic Eruption Based on Community Earth System Model Simulation’, Geophysical Research Letters, 47(18), pp. 1–10. doi: 10.1029/2020GL089416.
Liu, H.-Y., Lauta, K. C., & Maas, M. M. (2018). Governing Boring Apocalypses: A new typology of existential vulnerabilities and exposures for existential risk research. Futures, 102, 6–19. https://doi.org/10.1016/j.futures.2018.04.009
Mani, L., Tzachor, A. and Cole, P. (2021) ‘Global catastrophic risk from lower magnitude volcanic eruptions’, Nature Communications. Springer US, 12(1), pp. 8–12. doi: 10.1038/s41467-021-25021-8.
Papale, P., and Marzocchi, W. (2019) ‘Volcanic threats to global society’, Science, pp. 1275–1276. doi: 10.1126/science.aaw7201.
Papaple, (2018) 'Global time-size distribution of volcanic eruptions on Earth' Scientific Reports, 8:6838 | DOI:10.1038/s41598-018-25286-y
McConnell, J. R. et al. (2020) ‘Extreme climate after massive eruption of Alaska’s Okmok volcano in 43 bce and effects on the late roman republic and ptolemaic kingdom’, Proceedings of the National Academy of Sciences of the United States of America, 117(27), pp. 15443–15449. doi: 10.1073/pnas.2002722117.
Miller, G. H. et al. (2012) ‘Abrupt onset of the Little Ice Age triggered by volcanism and sustained by sea-ice/ocean feedbacks’, Geophysical Research Letters, 39(2). doi: 10.1029/2011GL050168.
Newhall, C., Self, S. and Robock, A. (2018) ‘Anticipating future Volcanic Explosivity Index ( VEI ) 7 eruptions and their chilling impacts’, 14(2), pp. 572–603. doi: 10.1130/GES01513.1.
Nooren, K. et al. (2017) ‘Explosive eruption of El Chichon volcano (Mexico) disrupted 6th century Maya civilization and contributed to global cooling’, Geology, 45(2), pp. 175–178. doi: 10.1130/G38739.1.
Ord, T. (2020) ‘The Precipice: Existential Risk and the Future of Humanity’,
Osipov, S. et al. (2021) ‘The Toba supervolcano eruption caused severe tropical stratospheric ozone depletion’, Communications Earth & Environment. Springer US, 2(1), pp. 1–7. doi: 10.1038/s43247-021-00141-7.
Rougier, J. et al. (2018) ‘The global magnitude–frequency relationship for large explosive volcanic eruptions’, Earth and Planetary Science Letters, 482, pp. 621–629. doi: 10.1016/j.epsl.2017.11.015.
Self, S. (2015) ‘Explosive Super-Eruptions and Potential Global Impacts’, in Volcanic Hazards, Risks and Disasters, pp. 399–418. doi: 10.1016/B978-0-12-396453-3.00016-2.
Sparks, S. et al. (2005) Super-eruptions: global effects and future Threats, English. Available at: https://www.researchgate.net/publication/37145367_Super-eruptions_global_effects_and_future_threats (Accessed: 11 October 2021).
Swindles, G. T. et al. (2018) ‘Climatic control on Icelandic volcanic activity during the mid ‑ Holocene’, (1), pp. 47–50.
Westaway, K. E. et al. (2007) ‘Initial speleothem results from western Flores and eastern Java, Indonesia: were climate changes from 47 to 5 ka responsible for the extinction of Homo floresiensis?’, Journal of Quaternary Science. John Wiley & Sons, Ltd, 22(5), pp. 429–438. doi: 10.1002/JQS.1122.
Wilcox, B. H. et al. (2015) Defending Human Civilization from Supervolcanic Eruptions, California Institute of Technology.
Xu, C. et al. (2020) ‘Future of the human climate niche’. doi: 10.1073/pnas.1910114117.
Thanks so much for writing this. There are a lot of new leads on understanding the risks here, and it is great to see people working on what I think is the largest of all the natural risks.
I'd be very interested to see what estimates of the overall existential risk from supervolcanic eruptions you come up with. As direct extinction appears particularly difficult, much of the question comes down to estimates of the chance that an eruption of a given magnitude would cause the collapse of civilisation and the chance of recovering from that collapse. Factoring the risk in this way may be helpful for both showing where disagreements between researchers lie and for understanding where the current uncertainty is.
Note also that there was a numerical mistake in my supervolcanic eruption section which I explain on the book's Errata page: https://theprecipice.com/errata
Thanks for this Toby. I like your suggestion about factoring the risk in this way, and we'll keep you informed about where this all leads. Regarding civilisation collapse & recovery, there's certainly a lot of parallels to abrupt cooling from nuclear and asteroid winters, though the nearer-field hazards (and resulting cascading impacts), may be significantly different. One major uncertainty in this seems to be the location of a super-eruption, which will strongly dictate its effects on society, e.g. similar magnitude super-eruption occurring in the Mediterranean versus New Zealand. So one of the things we hope to look into is identifying the regions & volcanoes where the next super eruptions are most likely to occur. Your book & the longtermism concepts have certainly made me reassess what the most important questions in our field are!
Thanks so for taking the time to write this up! I've been (casually) curious about this topic for a while, and it's great to have your expert analysis.
My main question is: How tractable are the current solutions to all of this? Are there specific next steps one could take? Organizations that could accept funding or incoming talent? Particular laws or regulations we ought to be advocating for? Those are all tough questions, but it would be helpful to have even a very vague sense of how far a unit of money/time could go towards this cause.
Not sure how others will respond, but just to offer one data point: this was really surprisingly high to me.
The only thing that jumps to mind is Luisa Rodriguez's work on famines during a civilizational collapse or nuclear winter: https://forum.effectivealtruism.org/posts/GsjmufaebreiaivF7/what-is-the-likelihood-that-civilizational-collapse-would
Not quite the same, but as you mention, "the closest analogy is nuclear war scenarios". They feel similar in that the worst case scenarios seem to be various hard to predict follow-on effects, e.g. there's a resource shortage, people panic and chaos ensues.
Minor nit: As I understand the term's usage, the events you describe would probably not entirely qualify. One org describes "s-risk" as "risks of cosmically significant amounts of suffering". A few other things I've read focuses on really astronomically large (in terms of population or timescale) almost science-fiction-esque scenarios, for example, colonizing the galaxy, producing 10^50 humans, and then torturing them all for a trillion years. But I'm not 100% confident that's the canonical definition, so your usage might be totally fine.
Thanks for your input!
Yes we think there are tractable solutions to reduce the impact from these large eruptions, and we're currently planning these behind the scenes. The reason for this in part is that there has been little done to model & understand consequences of such eruptions, most of the work in the disaster risk focuses on the more frequent volcanic risks. There's no organisation that's currently looking at these extreme hazards in a global sense, so work to coordinate and focus the volcano community (in a similar way to the asteroid community some decades back), could be really effective (again something we're starting to think about). We'll do some fuller cost-benefit analysis, but very little (if any) funds and time are being put into extreme volcanic risk reduction, yet the financial losses may be substantial (~trillions). Sorry if that's a bit vague-we're just starting to think about this.
Thanks for this- we'll read this post with interest.
I think you're right here (I was struggling with some of the definitions), so I've removed the bit about 's-risks'.
Thanks again!
Exciting to hear about your upcoming plans, thanks!
As an overarching review of geospatial data and geoscientists role in EA, I wrote a post and started a channel role-geoscientists on EA anywhere. I would love to see people interested in contributing towards geospatial X-risk here: https://forum.effectivealtruism.org/posts/JaACyD72oacQB9WDn/more-than-earth-warriors-the-diverse-roles-of-geoscientists
Great post!
To reiterate what AppliedDivinityStudies said, I would love to hear more about proposed solutions to this problem. For example, what do you think of this paper on preventing supervolcanic eruptions?
Interventions that may prevent or mollify supervolcanic eruptions
Thanks!
As the authors put it in that paper:
I think this is right, and until we can competently model how a magma will respond to any interventions that we might do, it's perhaps too risky to do at the moment. Nevertheless volcanologists have gone the other way and completely dismissed this whole concept of intervention. Personally, I think it be very worthwhile to investigate this concept in the lab and with numerical models, as after all, humans have drilled directly into magma reservoirs by mistake (while looking for geothermal energy) (~4 times in fact!) with limited negative consequences. So the knowledge we can find out by drilling magmas (one of the links I shared in the conclusions) would be highly informative for the coming decades of volcano science.
At the moment, there are far less risky options that could do to mitigate the risk in the short term, for instance we haven't even identified all the volcanoes capable of climate-altering eruptions, and how we can best to monitor them (many of these will not even be monitored, especially in resource-poor, volcano-rich countries like Indonesia and the Philippines).
One of the authors here - yes there is risk trying to prevent an eruption. Lower risk and providing protection against many other catastrophes than volcanic is preparing to scale up resilient foods quickly. It is also more cost effective.
Hi David, yes totally agree and meant to add this to my answer above. In fact, I think our post only strengthens the case for looking into resilient foods.
Great post and answers I really enjoyed it.
I am concerned about the volcanos that are not being monitored in developing countries. I actually live in a vulcano that is very likely to not be properly monitored (Mt. Cameroun) and that has had activity in recent decades.
Is there anything that small NGOs, local Universities, local governments, and civil society can do to help monitor vulcano activity? what risk mitigation measures can be realistically planned and promoted in low income countries?
Sorry if I am out of topic. I thought of writing privately but someone else might find the answer useful.
Thanks for this question MarcSerna.
Unfortunately, the lack of monitoring isn't limited to low-income countries, and even countries like the US struggle to maintain monitoring networks due to financial constraits (and changes in office). On increasing our monitoring capacity, the use of satellite technology is greatly enhancing our capabilities but we still have a long way to go for this to be a robust method. Often we must request satellites be flown over volcanoes, rather than it being routinely conducted and imagery can also be expensive. It should also be noted that volcanoes don't necessarily show signs of imminent activity. Some eruptions occur with minimal to no precursory activity (tremors, ground deformation etc.) so monitoring and prediction cannot always be a definite science.
One way that to be able to build our capacity for volcano monitoring would be through community empowerment, essentially building awareness within communities about how to identify signs of change at volcanoes and being able to report their concerns. Many citizen science projects are aiming to explore just this.
For now, I believe education and outreach with at-risk communities is essential to ensure preparedness of those most exposed. Certainly, my experience with the recent La Soufriere eruption in St Vincent was able to demonstrate the efficacy of long-term education programmes, resulting in no direct loss of life. Of course, dealing with disasters is multi-faceted and preparedness should also be directed at local authorities and stakeholders to ensure their preparedness to respond to a crisis. Again though, there is a severe funding source lack for these programmes and governments often do not prioritise this.
Thank you! Are there any public educative materials you recommend as a base?
Hi Marc,
Clive Oppenheimer's, 'Eruptions that shook the world' is a great introduction to volcanoes and their role on society. Cheers!
This is pretty long. Is there something like an abstract or executive summary of the post? Skimming a few of the expected places didn't feel like I was quite getting that without reading the whole thing.
Hi Gordon, I think by reading the 'challenging assumptions and why we think the current risk may be underappreciated' and 'Conclusions and the future' sections, you'll get a summary of most of the main points.
Thank you, Mike and Lara, for posting this analysis of the threat that large scale volcanic activity poses. This is rarely, if ever, covered in the media, and then only for a day or two when a specific eruption is occurring. I have long observed that this is not being taken seriously. We hear constant hysterical reporting about the possible effects of climate change (of which we still do not know enough about), but nothing about the absolute certainty of a major volcanic eruption taking place.
I have read that there seems to be some correlation between grand solar minimums and increasing volcanic activity. Have you come across any research on this?
Thanks and Regards,
Ken
Thanks Ken! Glad you appreciate the importance of this topic.
I'm afraid I've not come across much research about the potential correlation between grand solar minimums and volcanic activity, but let me know where you've come across this and I can look into it.