260 million years ago, our planet had an unfamiliar geography. Nearly all of the landmasses were united into a single giant continent known as ‘Pangea’ that stretched from pole to pole. On the other side of the world you would find a vast ocean, even larger than the present Pacific, called Panthalassa. The Pangean era lasted 160 million years, and 80 million of these were extremely inhospitable to animal and plant life, coinciding with two mass extinctions and four other major extinction events. This is why Paul Wignall, a Professor of Palaeoenvironments at Leeds has called the Pangean era ‘The Worst of Times’. Understanding why the Pangean era was so miserable helps inform several questions of interest to those studying existential risk.
● What level of natural existential risk do we face now, and have we faced in the past?
● What is the threat of super-volcanic eruptions?
● How much existential risk does anthropogenic climate change pose?
1. Background
There have been five mass extinctions so far. The Ordovician–Silurian (450-440 million years ago) and the Late Devonian (375-360 million years ago) each preceded the age of Pangea. The Pangean period coincided with the two worst mass extinctions, the huge Permian-Triassic mass extinction (252 million years ago) and the Triassic-Jurassic extinction event (201 million years ago).[1] The last crisis, the Cretaceous–Paleogene event (65 million years ago), accounted for the dinosaurs and occurred once continental drift had done its business and Pangea had broken apart. With the exception of the end Cretaceous extinction, since the breakup of Pangea, it has been relatively plain sailing for Earth’s various species, until humans started killing off other species themselves.
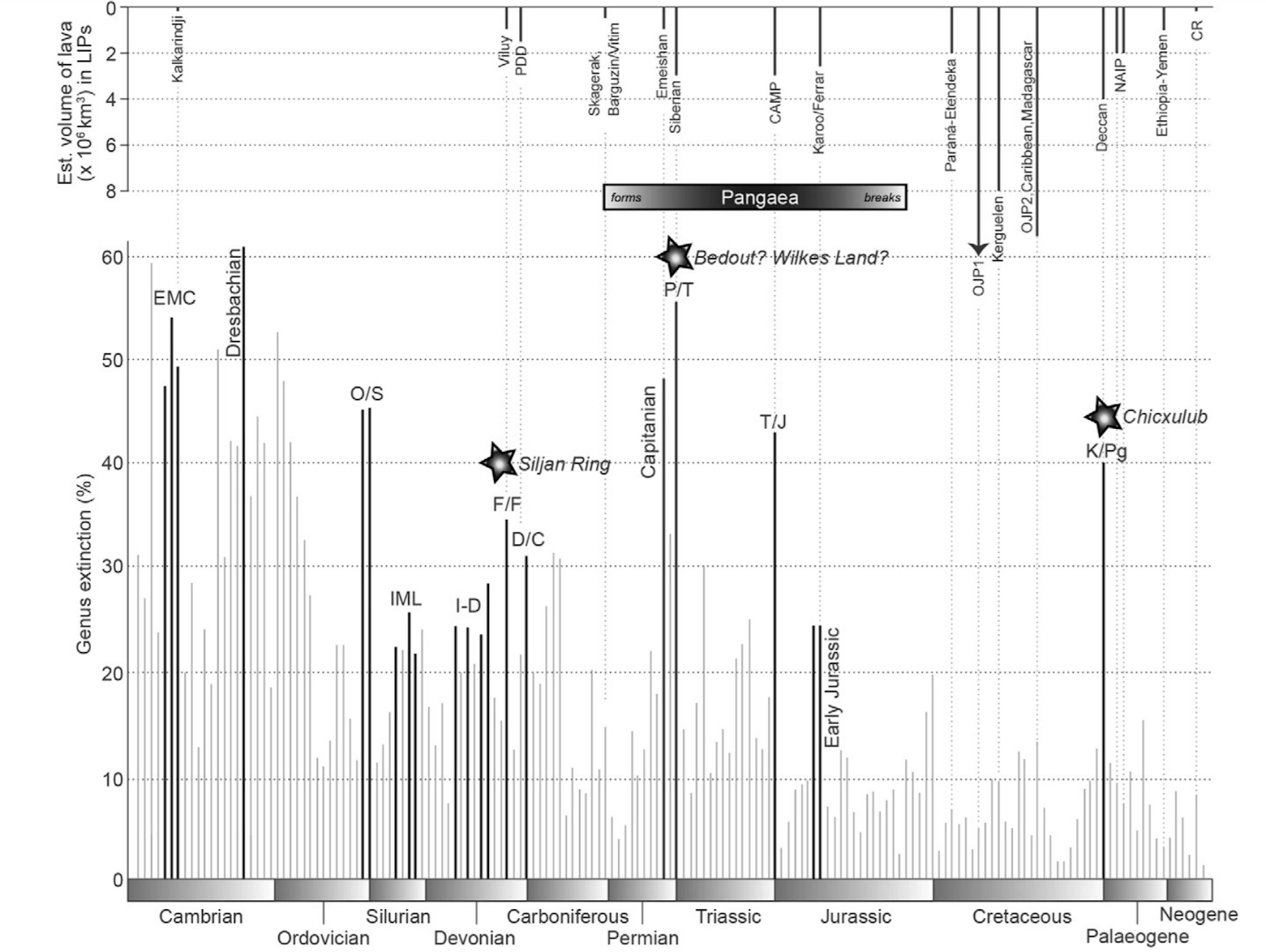
As one can see on this diagram, in the 145 million years since the start of the Cretaceous, the average rate of global genus extinctions from extinction events has been around 5% and never passed 15%, except for the death of the dinosaurs. But in the 80 million years from the first Pangean extinction event, the Capitanian, to the early Jurassic extinction events, the average rate of global genus extinctions in extinction events is more around 15-20%, and 12 events produced global genus extinction rates in excess of 15%.
Below is a useful chart from Wikipedia on the Phanerozoic, which shows the long-term trend in biodiversity as well as the impact of different extinction events.
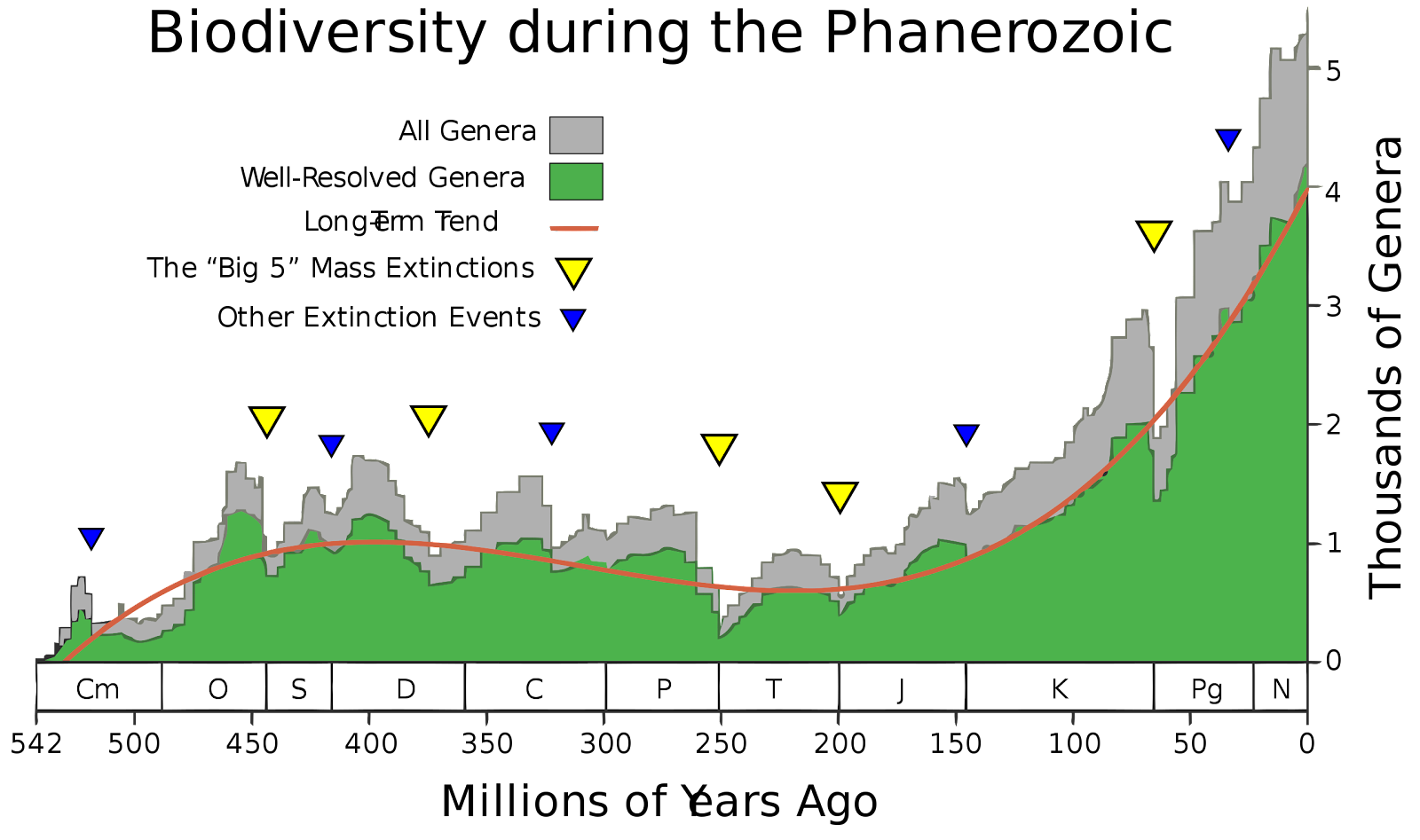
Again, this highlights how unusually bad things were in the Pangean era - specifically the 80 million years after the Capitanian extinction event 260 million years ago. But it also highlights how good things have been since the end of the Pangean era and the start of the Cretaceous (145 million years ago).
2. What caused such ecological trauma in Pangea?
Huge volcanic eruptions were implicated in all of the six major extinction events in the Pangean era. One can see this in the first diagram above, where the volcanic eruptions are shown at the top and the line traces down to corresponding extinction events at the bottom. Every Pangean extinction event coincided with the outpouring of enormous fields of lava that, once cooled, produced what geologists call Large Igneous Provinces (LIPs).[3]
To put these LIPs in context, the eruption of Mount Pinatubo in 1991 produced 10 cubic km of magma, which caused the Earth to cool by about half a degree. The eruption of the Siberian Traps which appeared to cause the end Permian extinction produced 3 million cubic km of magma. You can see the volume of magma for all major LIPs at the top of the first diagram above.
These volcanic eruptions emitted sulphur dioxide, carbon dioxide and halogen gases, each of which could potentially have an effect on the ecosystem. Bond and Grasby provide a useful diagram of the kill mechanisms for the Permian extinction, the greatest ecological disaster of all time.
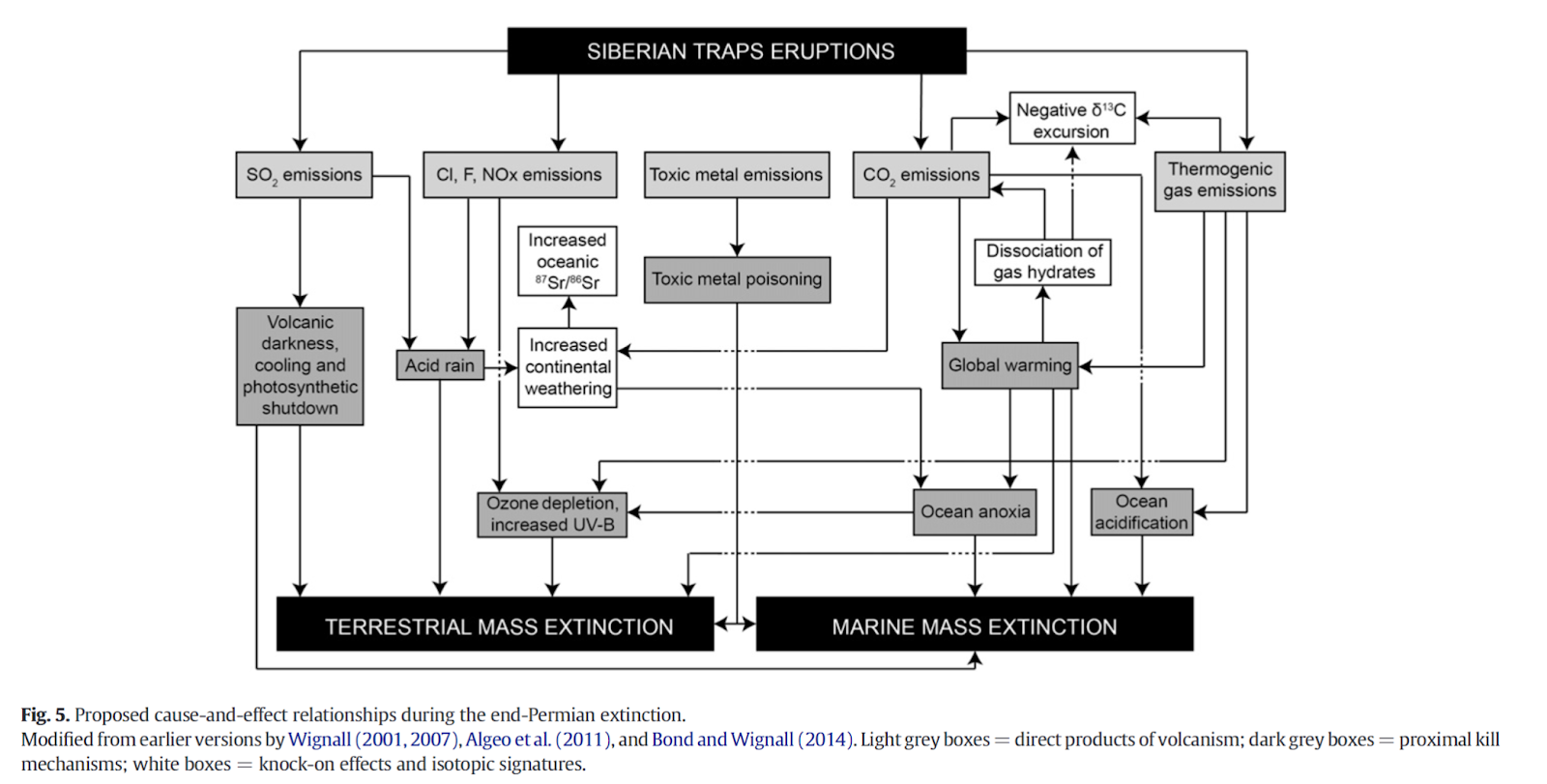
A single pulse of sulphur dioxide into the atmosphere can cause cooling for two to three year, after which time it is usually rained out. Thus, sulphur dioxide is probably only capable of driving death-by-cooling if eruptions were frequent and of high volume and were sustained for several centuries at a time. Unfortunately, the geological record of LIPs is not sufficiently resolved to permit an evaluation of whether this has actually happened during a mass extinction interval.[5]
In contrast, a single pulse of CO2 can cause warming on geological timescales: about a fifth of CO2 stays in the atmosphere for several thousand years after it is injected.[6] The total CO2 release from a LIP, such as the Siberian Traps has been estimated at 30,000 billion tonnes, ten times the mass in today's atmosphere,[7] and three orders of magnitude greater than current annual emissions.
The release of such massive amounts of CO2 could cause extinctions through several mechanisms:
● Warming places thermal stress on organisms
● Warming reduces the capacity of the ocean to absorb oxygen, which can lead to ocean anoxia.
● CO2 dissolves in the ocean causing ocean acidification, which can lead to marine extinctions.
● CO2 can build up in tissues, a process known as hypercapnia, which Knoll has argued contributed to extinctions.[8]
The Pangean world was generally very hot and arid, with atmospheric CO2 at 3,000ppm[9] (compared to around 400ppm today). Average ocean temperatures before the end Permian disaster were 20°C and peaked at 40°C in the early Triassic. At no point did temperatures drop below 32°C in the 5 million years after the end Permian event.[10] For comparison, modern equatorial sea temperatures average around 28°C and never exceed 30°C. Peak temperatures in the early Triassic were the same as you would find in a bowl of very hot soup.[11]
3. Volcanism in the post-Pangean era
The diagram pasted above shows that:
Massive LIPs + Pangea = major extinction.
In Pangea, every time there was massive volcanism, there was a major extinction.[12]
However, once we remove Pangea from the equation, we no longer get a major extinction. Since the end of Pangea, comparably massive volcanism has barely registered on the fossil record.[13] 135 million years ago, an eruption went to form one of the largest LIPs - the Paraná-Etendeka Province. And yet, the eruption caused neither catastrophic environmental change nor mass extinction.[14]
The most interesting LIP for modern climatologists is the North Atlantic Igneous Province, which, according to Wignall, produced huge amounts of lava, even by LIP standards.[15] This produced 70,000 years of intense global warming, causing the Paleocene-Eocene Thermal Maximum, which is the closest geological analogue for the kind of global warming that humans are set to produce. During the Paleocene Eocene Thermal Maximum, global temperatures rose by ∼5°C in 10,000 years with average values in northern tropics estimated to have been ∼31–34°C.[16] However, during the Paleocene Eocene Thermal Maximum, losses were very minor and on the whole, this was a time of flourishing and success for a broad range of animals.[17] The Eocene was very hot compared to today, but the name Eocene comes from the Ancient Greek ἠώς (ēṓs, "dawn") and καινός (kainós, "new") and refers to the "dawn" of modern fauna that appeared during the epoch.
One possible exception to this is that the Deccan Traps coincided with the death of the dinosaurs. But then of course this also coincided with a meteorite impact in Chicxulub, Mexico, complicating cause and effect.[18] Indeed, I think Wignall’s arguments in The Worst of Times lend support to the meteorite explanation because it is unclear why massive volcanism would have accounted for the dinosaurs, but have been otherwise so insignificant from the Cretaceous (145 million years ago) onwards.
Overall, the post-Pangean era is much more robust and resilient than the Pangean era. Indeed, the picture we see in our post-Pangean era is one of climate change - both cooling and warming - having little effect on species extinctions.[19] This is true for when the magnitude of warming was comparable to what we are in for in the next 200 years, and when, on a regional basis, the rate of warming was comparable to what we are in for in the next 200 years.
4. Why has the post-Pangean era been so benign?
Wignall argues that Pangea was so inhospitable because various carbon cycle feedbacks were not in play due to the unique geography and geology of the supercontinent.[20] By the early Cretaceous, the world was much more efficient at removing CO2, with the result that atmospheric CO2 concentrations were a tenth of the Pangean level.[21] There are several reasons for this:
● Rainfall weathering - In a supercontinent, huge areas are too far away from the sea to receive much rain, which reduces the scope for removal of CO2 by rainfall and weathering.
● Limestone deposition - In Pangea, limestone deposition - which sequesters CO2 in the oceans - was at a minimum because the shelf fringe of the supercontinent is much smaller than the shelf fringe of a collection of much smaller continents.
● The evolution of coccolithophorids - Coccolithophorids appeared in the late Triassic and help to sequester carbon because they use CO2 in shell formation and then sink to the bottom of the ocean when dead, which also helps to counteract ocean acidification.
● Terrestrial plants - The end Permian led to a mass extinction of terrestrial plants. Without plants, the weathering feedback still occurs, but plants make it happen much more rapidly. A world without plants is therefore much more prone to rapid climatic fluctuations.[22]
● Slow circulation in Panthalassa - Ocean circulation in the massive Panthalassa ocean would have been relatively sluggish, contributing to extensive subsurface oxygen shortage.[23]
Wignall provides the following diagram of the various processes of carbon sequestration:
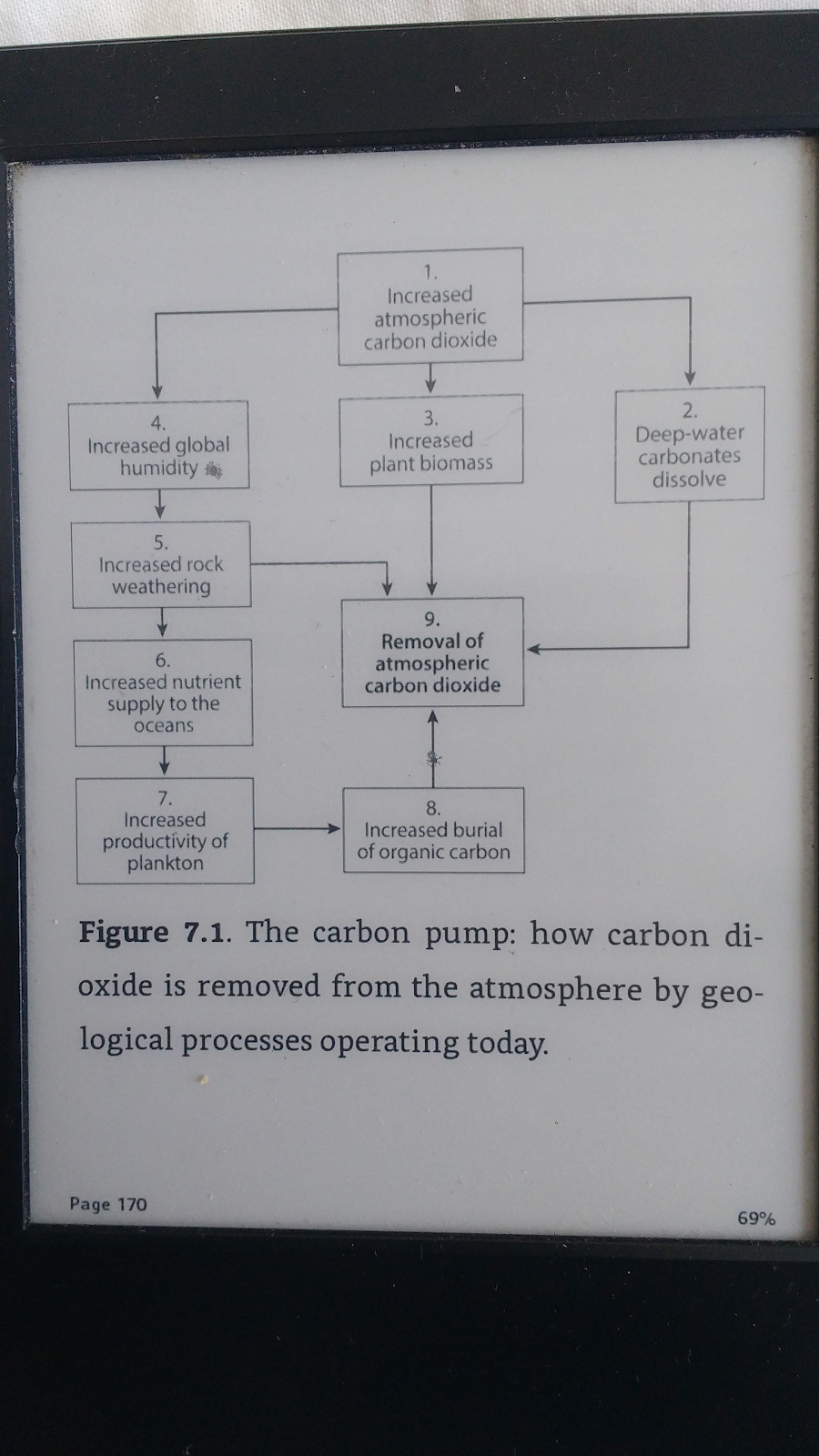
5. Possible lessons
The hypothesis that Pangea was uniquely ecologically vulnerable has many potential lessons for existential risk today.
Natural existential risk
Researchers have recently tried to place a bound on the natural existential risk that we face today.[24] The arguments here suggest that the level of natural existential risk is dependent on our geology. Past mass extinctions may not be a reliable guide to the extinction risk we face today and estimates of the risk may be biased upwards.
Risk from super-volcanic eruptions
[Edit - the numbers are wrong here]
In The Precipice, Toby Ord argues, using estimates from volcano researchers, that the existential risk from supervolcanic eruptions this century is 1 in 10,000,[25] with the posited mechanism being sustained global cooling. I am not sure to what extent these estimates are dependent on inferring the risk from past episodes of volcanism, including in the Pangean era. If they are, the estimate of the risk from volcanoes could be too high. Indeed, if the risk were this high, homo sapiens would have had to have gone through this trauma twice and survived at much lower levels of technological sophistication than today.
Moreover, the geological record suggests that volcanoes are more likely to have caused extinction via warming rather than cooling.
Risk from climate change
Some researchers have argued that CO2 release from volcanism is a worrying analogue for contemporary anthropogenic CO2 emissions. For example, Penn et al argue that plausible emissions scenarios predict a magnitude of upper ocean warming by 2300 that is 35 to 50% of that required to account for most of the end-Permian extinction intensity. The historical analogue lends weight to the idea that anthropogenic warming might cause a mass extinction.
If Wignall’s argument is right, we should be less worried about this analogy. The threat the ecosystem faced In the Pangean era was peculiar to the geography of Pangea and has receded since the fracturing of the supercontinent. Mercifully, our ecosystem is now more resilient than it once was.
6. Caveats and uncertainties
Several caveats and uncertainties should be noted:
● The context in which warming is occurring today is importantly different to that since the breakup of Pangea. Warming today is occurring in the context of widespread habitat loss and other environmental stress.
● There is vigorous scholarly disagreement about the causes of past extinction events.[26]
● I am unsure to what extent Wignall’s argument commands assent in the wider literature.
● Given the uncertainties involved in studying events so far in the past, it would not be at all surprising if current hypotheses were rejected in the coming decades.
[1] P. B. Wignall, The Worst of Times: How Life on Earth Survived Eighty Million Years of Extinctions (Princeton: Princeton University Press, 2015), 165.
[2] David P. G. Bond and Stephen E. Grasby, “On the Causes of Mass Extinctions,” Palaeogeography, Palaeoclimatology, Palaeoecology, Mass Extinction Causality: Records of Anoxia, Acidification, and Global Warming during Earth’s Greatest Crises, 478 (July 15, 2017): 14, https://doi.org/10.1016/j.palaeo.2016.11.005.
[3] Wignall, The Worst of Times, 9.
[4] Bond and Grasby, “On the Causes of Mass Extinctions,” 10.
[8] Andrew H. Knoll et al., “Skeletons and Ocean Chemistry: The Long View,” Ocean Acidification, 2011, 67–82.
[9] Wignall, The Worst of Times, 168.
[13] P. B. Wignall, The Worst of Times: How Life on Earth Survived Eighty Million Years of Extinctions (Princeton: Princeton University Press, 2015), 9ff.
[14] Wignall, The Worst of Times, 153ff.
[15] Wignall, 161. However, note that this is at odds with the first Bond and Grasby diagram above. I am not sure what explains this discrepancy.
[16] K. J. Willis and G. M. MacDonald, “Long-Term Ecological Records and Their Relevance to Climate Change Predictions for a Warmer World,” Annual Review of Ecology, Evolution, and Systematics 42, no. 1 (2011): 270, https://doi.org/10.1146/annurev-ecolsys-102209-144704.
[17] Wignall, The Worst of Times, 161ff; Willis and MacDonald, “Long-Term Ecological Records and Their Relevance to Climate Change Predictions for a Warmer World,” 270ff.
[18] Wignall, 9.
[19] Daniel B. Botkin et al., “Forecasting the Effects of Global Warming on Biodiversity,” BioScience 57, no. 3 (March 1, 2007): 227–36, https://doi.org/10.1641/B570306; Kathy J. Willis et al., “4 °C and beyond: What Did This Mean for Biodiversity in the Past?,” Systematics and Biodiversity 8, no. 1 (March 25, 2010): 3–9, https://doi.org/10.1080/14772000903495833; Willis and MacDonald, “Long-Term Ecological Records and Their Relevance to Climate Change Predictions for a Warmer World”; Terence P. Dawson et al., “Beyond Predictions: Biodiversity Conservation in a Changing Climate,” Science 332, no. 6025 (April 1, 2011): 53–58, https://doi.org/10.1126/science.1200303.
[20] Wignall, The Worst of Times, chap. 7.
[23] Knoll et al., “Skeletons and Ocean Chemistry,” 13.
[24] Andrew E. Snyder-Beattie, Toby Ord, and Michael B. Bonsall, “An Upper Bound for the Background Rate of Human Extinction,” Scientific Reports 9, no. 1 (July 30, 2019): 1–9, https://doi.org/10.1038/s41598-019-47540-7; David Manheim, “Questioning Estimates of Natural Pandemic Risk,” Health Security 16, no. 6 (2018): 381–390.
[25] Toby Ord, The Precipice: Existential Risk and the Future of Humanity, 1 edition (Bloomsbury Publishing, 2020), 167.
Excellent post. I highly value such summaries of known and relevant factual information, and views on lessons we can learn. Thank you for putting this together.
I'm also curious what prompted you to look into this topic?
Minor: The in-text links to endnotes link to the page for making a new forum post, which presumably is not intended.
Minor: The following sentence right before section 4 starts seems jumbled?
I think the sentence does make sense, though it is certainly badly written!
Interesting post, thanks for writing it!
I think the "20 times" claim is incorrect, unless I'm misunderstanding you. Ord's estimate is per century, not per year. It seems the usual estimate of how long "homo sapiens" has been around is 200,000 years. So we'd have had to face a (constant) 1 in 10,000 per year risk ~20 times, but a 1 in 10,000 per century risk only ~0.2 times.
This doesn't detract from your other point about why Ord's estimate may be too high, though.
Ah yes - that's a good point, cheers!
Very interesting, thanks. I would just suggest adding to your list of caveats at the end that today's rate of warming is much faster than in episodes like the Paleocene-Eocene Thermal Maximum, which increases risks.